This
Page was Started Dec. 18,
2004
Updated 1-25-05, add link for CAD layout of SRD
board
Updated 2-25-11,
Update this page.
This page will act as a design guide for
building a frequency multiplier using a diode that has a long Carrier
Lifetime and short Transition Time. Some diodes that
exhibit these qualities are Step Recovery Diodes, Varactor Diodes, and
PIN Diodes. This page uses the terms SRD, Snap, and PIN
Diodes randomly, but they all mean the same thing. I did not
design
this multiplier for maximum efficiency. Had I done so, the input and
output would be specific (64 MHz X16 = 1024 MHz). The circuits and
components would be extremely critical, and the layout topology would
probably be impossible to duplicate. So, if you say "that doesn't look
like any multiplier I have ever seen", you are right. However, this
scheme will work with different frequencies and different
multiplication factors other than just 64 MHz and X16.
My original intention was to build a frequency
multiplier for the Modularized Spectrum Analyzer. The MSA's 2nd
Local
Oscillator, which runs at 1024 MHz, is a PLL/VCO/Amplifier combination.
There is absolutely nothing wrong with that scheme, but it can be
replaced with
this SRD multiplier scheme to improve Spectrum Analyzer phase noise
results. The total SA phase noise improvement is only about 1 dB.
Therefore, using a multiplier scheme for LO 2 has no benefit unless the
SA's LO 1 is modified for low phase noise operation.
At the end of this page is some technical
information for Step Recovery Diodes.
Go back to the MSA Main Page
Frequency Multiplier Block Diagram to
replace PLL2 and VCO
2:
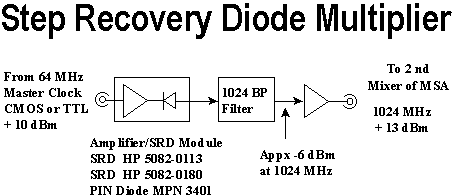
This frequency multiplier scheme consists
of a driver, impulse generator (Snap Diode), filter, and an amplifier.
The filter is a coaxial cavity filter tuned to 1024 MHz and an
insertion loss of 1.5 dB. The amplifier
has approximately 20 dB of gain and is saturated with an output of
about +13 dBm. In the following diagram, the driver and impulse
generator are combined onto one pwb.
The Driver, the Step
Recovery Diode Module
This module can respond to a sine input, but it
is expected to receive the
square CMOS signal
from a 64 MHz Master Clock. The
first section of the 74AC04 (or ACT04) will convert the input.
The second
stage will square up the signal. The third stage is a
parallel
driver, to assure maximum
drive to the Snap Diode. The Snap Diode
acts as the impulse generator. Notice that the Snap
Diode is
not a Step Recovery Diode, but rather, a PIN
diode (MPN
3401). Although not
as efficient as an SRD, this particular PIN diode performs well in this
design. It is also extremely cheap and available (about 50 cents,
compared to about $20 for an SRD). The completed multiplier
measures only 3 dB less efficient than when using the HP 5082-0113, an
SRD.
R3 is the self bias resistor
for the PIN. Although its value is shown to be 50 ohms, it will be
changed to optimize the circuit efficiency or accomodate different
SRD's. Notice, there is no load or resonator on the
output of D1. A resistive or inductive load must be shunted at J3 for
the diode to function. A 50 ohm load could be placed at J3 to create a
"Comb Generator". But for this application, the hairpin input of the
cavity filter will act as an inductive load for the SRD.
The actual measured output of the SRD
module (as a comb generator) is approximately -23 dBm at 1024
MHz. When
the resistive load is replaced with the band pass filter, the filter's
output power is -5 dBm at 1024 MHz. I realize that this sounds like
voodoo
reverse-conservation of energy, but the band pass filter
acts as a
reflector to all harmonics except the one at 1024. The reflected
harmonics are directed back to the SRD and their combined power will
increase
the efficiency of the SRD action.
The Snap Diode can be mounted in either
direction. I found that
maximum power output was obtained with the diode's cathode on the
output.
This is not due to the effeciency of the diode. It attributed to
the '04 driver, which has more current capability on its negative edge
than
its positive edge.
Changing the bias resistor will change
the length of time that reverse current is supplied to the diode. This
does not change carrier lifetime of the SRD, but it does change the
total circuit lifetime. This changes the circuit efficiency. Using
various resistor values, the
filter's output power can be maximized. The actual
resistor value depends on many factors, but it should be between 200
ohms
and 2000 ohms. After much "resistor tweeking", my final bias resistor
value was 1.1 K ohms.
Power could be further increased by using
a
specific length delay line between the SRD output and the input to
the cavity filter. A critical phase delay would allow the reflections
from the filter to affect the SRD efficiency. This delay line
technique could possibly result in a power output of
+6 dBm. However, this is below the requirements
for the mixers in the MSA, so a post amplifier is needed.
Without the delay line an output from the
filter might be as high as +2 dBm, but a more conservative expectation
is somewhere between -6 dBm and 0 dBm. Since an amplifier is required
for either topology (with or without delay line), I chose not to
include the delay line in the design. Its length is extremely critical,
anyway. An amplifier can increase the total power to drive any mixer.
Here are screen shots of the SRD Module
Board
Layout. I
used ExpressPCB software, which can be accessed by clicking, SRD Module PWB.
The size of the board is 1.4 x 1.35
inches (this does not conform to present SLIM requirements).
Layer 1 is the silkscreen, layer 2 is the top copper, layer 3 is the
bottom copper (as seen from top) :
Click for : all Layers,
Layers
2 and 3,
Layer 2,
The board is layed out so that either the DIP
or SOIC package 74AC04 can be used. I used the SOIC for testing.
The
Band Pass Filter
The following dual coaxial cavity filter can be used as the band pass
filter, to pass the 16 th harmonic of the SRD's 64
MHz input. I won't repeat the build process on this page.
For pictures and construction techniques, go to the Cavity Filter
page. This dual filter can be replaced with other narrow band filters
with good results. I have done extensive
multiplier testing using a single coaxial cavity filter
instead of this two stage coaxial cavity filter. The 15th and 17th
harmonics (960 MHz and 1088 MHz) are attenuated about -30 dB below 1024
MHz, which is adequite for use in the MSA.
1024 MHz Dual Cavity Filter,
dimensions are inches.